info page
Galactose
Health Hazard Galactose?
– A Scientific Analysis by Michael Nehls, MD. PhD (July 8, 2023)
Again and again, galactose (mucilage sugar) is touted as a supposedly intelligent miracle sugar for mental fitness; daily intake is said to offer health benefits, especially for Alzheimer’s disease. With such misleading promises, a lot of damage is done, but also a lot of sales are made. Yet a simple Google search of the words galactose & Alzheimer’s & NCBI (National Center for Biotechnology Information, the U.S. database for medical publications) yields pages and pages of publications in which galactose is used to induce Alzheimer’s in animal models. This is exactly the opposite of the protective effect promised by galactose consumption in the relevant guides. Education is urgently needed.
Hippocampal Insulin Resistance
The starting point of Alzheimer’s dementia is the autobiographical memory center, anatomically called the hippocampus because of its seahorse-like structure. In contrast to the rest of the brain, this ancient region of the brain (archicortex) protects itself from fluctuations in blood glucose levels by protecting the supply of glucose (dextrose or blood sugar) through an insulin-dependent transport system. This protective mechanism can be compromised by our modern, unbalanced lifestyle. Hippocampal insulin resistance develops.[1] As a result, the hippocampus can virtually no longer absorb sugar, which can be used diagnosti-cally for early detection of the disease. An imaging technique called FDG-PET/CT is used for this purpose. Glucose labeled with a radioactive fluorine isotope (18F-fluorodeoxyglucose, 18F-FDG) is inject-ed and absorbed by body cells like “normal” glucose. This accumulation in organs and tissues can be visualized using positron emission tomography (PET) in combination with computed tomography (CT).[2] Typically, FDG-PET/CT shows reduced glucose uptake in the hippocampal region of the brain at a very early stage of Alzheimer’s disease, usually years before the onset of clinical Alzheimer’s symptoms. This is referred to as neuronal or type 3 diabetes, but this is a misnomer because this local insu-lin resistance does not result in sweet urine as in type 1 or type 2 diabetes mellitus. Hippocampal insulin resistance is not diabetes mellitus, although diabetics have a higher risk of developing Alzheimer’s disease. (However, people with other diseases of civilization also have a higher risk, since the disease processes often have the same causes and reinforce each other).
The causes of hippocampal insulin resistance are many. Among them are chronic inflammation (including AGEs, see below)[3] ,permanently high levels of stress hormones, trans fatty acids and last but not least the Alzheimer’s toxin, i.e., β-amyloid, which forms neurotoxic oligomers (as a monomer it ensures the stability of new memories). Against chronic stress is a productive adult hippocampal neurogenesis a prerequisite, i.e. a lifelong production of new neurons in the hippocampus, because it ensures, among other things, a high psychological resilience.[4] Productive adult hippocampal neurogenesis is also the best protection against Alzheimer’s dis-ease or its dysfunction, the primary cause of this hippocampal dementia, as I showed in a 2016 review.[5] Adequate deep sleep and physical activity ensure that β-amyloid is broken down at night (so that it does not build up to high concentrations where it sticks together to form the toxic oli-gomers).[6] Reducing ─ or better yet, eliminating ─ the intake of trans fats, and generally eating a diet that does not cause chronic inflammation, also protects against the development of hippocampal insulin resistance. In other words, it is consistently lifestyle factors that cause or prevent us from developing hip-pocampal insulin resistance and Alzheimer’s disease in the long run.
If the insulin-resistant hippocampus were offered only glucose as an energy source, which is unfortunately the norm in modern Western diets, it would literally starve to death, even with high blood glucose levels. This inadequate glucose uptake in the presence of unnatural hippocampal insulin resistance is now used as an argument for providing the hippocampus with galactose as an alternative energy source, since it can be absorbed independently of insulin. However, apart from the fact that the high intake of galactose required is as unnatural as the lifestyle that is usually responsible for hippocampal insulin resistance, this approach is expensive, ineffective and highly unhealthy – not to mention that there is a much cheaper, actually healthy and natural alternative.
Galactose ─ Casting Out The Devil With Beelzebub
To ensure a steady supply of energy to nerve cells, it is important to avoid energy fluctuations. Almost everyone has experienced the unpleasant sensation of low blood sugar: rapid pulse, cold sweat, feeling pale, restless and nervous, trembling, weak knees and ravenous appetite. Diabetics know that high blood sugar, which causes the brain to swell (the neocortex absorbs sugar without insulin regulation), is also very unpleasant ─ in both cases you get scared, and not without reason, because coma is imminent. Since our brain cannot store sugar, glucose must be constantly supplied, and therefore blood glucose levels are regulated by insulin within very narrow limits. Galactose bypasses this regulation, which seems to be an advantage in terms of hippocampal insulin resistance. However, the opposite is true: each intake results in an unregulated rise and subsequent fall. Hippocampal hyperglycemia and subsequent hypoglycemia occur. While there is no such thing as a hippocampal coma, this is unnatural and certainly not healthy. Only during breast-feeding do infants receive galactose through breast milk, i.e. in a natural way, but combined with glucose in the form of lactose (milk sugar). The release of the galactose portion is regulated by cleavage by the enzyme lactase. In most infants worldwide[7], lactase production stops after breastfeeding because it is complete-ly unnatural to consume breast milk or ruminant milk after that. As a result, they are lactose intolerant for the rest of their lives and must avoid non-fermented dairy products to prevent further lactose intake.
Because artificially supplied galactose is completely unregulated after intestinal absorption, there is initially a galactose spike in the blood (which would be missed by blood glucose moni-toring, which only measures glucose). Such sugar spikes are very unhealthy because galactose is a reducing sugar that chemically reacts with the free amines of amino acids in proteins. A Schiff base is formed, a compound that is easily oxidized and then very stable. The resulting chemical compounds are called advanced glycation end products (AGEs), which you can think of as a sugar-coated table surface on which you spilled a sweet drink, except that it is no longer easy to clean up. AGEs are not only biomarkers, but also a major cause of accelerated aging (which is conveniently what the acronym refers to). AGEs are foreign bodies that our immune system tries to remove, which is not easy due to their atomic stickiness. There is even a specific receptor for them, abbreviated RAGE (coincidentally, meaning angry). Activation of RAGE by AGEs is actually an “angry” response that causes chronic inflammation via various signaling pathways, including the generation of reactive oxygen species (ROS), which together accelerate the aging process and thus are a cause of hippocampal insulin resistance (see above).[8]
Galactose In Animal Models Of Accelerated Aging And Alzheimer’s Disease
Thus, galactose causes hippocampal insulin resistance in animals, which is exactly what the rec-ommendation to humans to consume galactose is trying to overcome.[9] Galactose has also been shown in animal models to inhibit adult hippocampal neurogenesis and even cause hippocampal neurodegeneration, resulting in chronic memory loss.[10] This property of galactose is also used in animal models to experimentally induce Alzheimer’s disease.[11] In both mice and rats, a daily dose of 0.1 gram (g) per kilogram (kg) of body weight, administered over a period of only a few months (usually injected into the abdomen), is sufficient to induce Alzheimer’s disease. According to a scientific article: ”Aging is a complex natural phenomenon characterized by de-generative changes in the structure and function of cells and tissues. D-galactose-induced aging mice are an artificial accelerated aging model that induces memory and learning deficits, oxida-tive stress, and neuroinflammation.”[12] This daily galactose intake would be equivalent to about 7 g of galactose in a 70 kg human (al-ready contained in 0.3 to 0.6 liters of milk; 3.5 g lactose or 1.75 g galactose per 100 ml). It has now been shown that these results from animal experiments can at least tend to be trans-ferred to humans: for example, the risk of Alzheimer’s disease increases in proportion to the (unnatural) consumption of milk by people who have outgrown infancy.[13] So why, you may ask, do some people recommend the consumption of galactose, especially when there is a healthy alternative?
A Health-Promoting Alternative ─ Inexpensive And Natural
When I was in medical school, I learned that glucose is the primary source of energy for our brain. But that’s not true, because there is an alternative to sugar that provides a steady supply of energy to our autobiographical memory center, even in the presence of pre-existing hippo-campal insulin resistance. These are the so-called ketone bodies. These have an even higher energy value than sugar (more energy released per unit of oxygen consumed) and are produced when saturated fatty acids are broken down in the liver after they have been mobilized from the fat stores by overnight fasting. Ketone bodies include acetoacetate (AcAc) and D-β-hydroxybutyrate (βOHB). These small molecules, unlike long- and medium-chain fatty acids, cross the blood-brain barrier very efficiently and do not require insulin to enter neurons and provide them with energy. Even in early childhood, when “baby fat” is broken down during long periods of sleep, the ketone bodies produced serve to provide energy for the growing brain. Not only do they provide a large portion of the brain’s energy needs, but their hormonal properties pro-mote neurogenesis and brain growth.[14]
Even in adults, ketone bodies are optimal energy suppliers and, as hormonal signaling agents, activate adult hippocampal neurogenesis.[15] Since they also accelerate the degradation of dys-functional, aged cell organelles, they are a true fountain of youth for our brain. In 2016, the Nobel Prize in Medicine was awarded for the discovery of the health-promoting or rejuvenating effects of fasting.[16] With their multiple functions, ketone bodies ensure the growth, rejuvena-tion or[17] regeneration and energy supply[18] of the hippocampus into old age, and are therefore a natural weapon against Alzheimer’s disease, as the tabular comparison with sugar (from my book “The Exhausted Brain”, p. 217) shows.
As the table shows, high levels of ketones signal the hippocampus to grow. They also activate nerve cell-protecting immune cells.[19] Elevated blood glucose levels, on the other hand, lead to inflammation, which inhibits brain growth and makes our neurons age faster. Ketone bodies, on the other hand, rejuvenate our brains by improving cell metabolism and activating the break-down of waste in our cells.
Fasting is therefore healthy because ketone bodies are healthy and protect us from many (mod-ern civilization) diseases. And fasting can be quite simple, because after only about 12 hours of sugar rest, ketone bodies replace more and more glucose, or blood sugar, as the primary energy source for our brain (see below). This period is roughly equivalent to our nightly sleep (plus for-aging in prehistoric times) and is therefore the most natural form of fasting. Hence breakfast literally means “breaking fasting”. However, as soon as we consume sugar in the form of bread, pasta, candy, or sugary drinks, the rise in blood sugar and subsequent release of insulin blocks the release of fatty acids from adipose tissue and thus stops the ketogenesis that occurs in the liver during fasting.
The evolutionary advantage of these hormonal effects could be seen in the fact that after a few hours of fasting, the organism usually feels the need to go in search of food. Thus, it experiences new things that a hippocampus with active neurogenesis is better able to store and process.
Ketones can be produced by the liver from mobilized fatty acids at rates of up to 150 g/day (150 g equals about 1500 kcal).[20] In humans, the basal serum level of βOHB, for example, is in the low micromolar range but rises to several hundred micromoles after 12 to 16 hours of fasting. [21] Importantly, when blood glucose is low, up to 60 percent of the brain’s energy needs can be met by ketone bodies, replacing glucose as the primary fuel.[22] Thus, ketones can continue to provide energy to the hippocampus even in the presence of insulin resistance-and protect us from Alz-heimer’s disease (and Alzheimer’s toxin) because of the hormonal properties mentioned above.[23] On the other hand, when we consume sugar, especially galactose, which is not regulat-ed by insulin, it has exactly the opposite effect.
However, ketones can continue to be produced efficiently after the fast is over, even if we do not mobilize long-chain saturated fatty acids from our fat stores. The trick is to get natural medi-um chain fatty acids from your diet. Unlike long-chain fatty acids, they are absorbed in the intes-tine and then transported directly to the liver via the portal vein, where they are metabolized into ketone bodies. Medium-chain fatty acids occur naturally in higher amounts almost exclu-sively in palm fruits such as coconut and in the milk of some mammalian species. Human breast milk, for example, has a particularly high concentration of medium-chain fatty acids, about ten percent.[24] Fatty acids specifically produced by the mammary gland during lactation promote fetal brain development.[25] From an evolutionary biology perspective, this was one of the key factors in the enormous brain development of humans.[26] Coconut oil naturally prolongs brain growth and regeneration throughout life, even protecting against Alzheimer’s disease.[27] If at this point you have become a little uncertain or nervous because the industry has told you that sat-urated fats are unhealthy, then I recommend my article “Coconut Oil is Pure Poison ─ A Scien-tific Refutation“.
So there is no need for brain-damaging galactose, but with the ketone bodies produced by the body there is an energy supplier with a hormonal protective function against aging processes and Alzheimer’s disease, which can not only supply our memory center with energy around the clock, but is also enormously cost-effective, as the following calculation shows:[28] To induce accel-erated aging, mice and rats are fed about 100 milligrams (mg) of galactose per kilogram of body weight daily, usually for only a few months. Extrapolated to an adult human of 70 kg body weight, this is about 7 g of galactose per day. This harmful amount is even less than the 10 to 12 g of galactose recommended for Alzheimer’s disease in an article in Natur&Heilen from April 2023.[29] 12 g of galactose per day (about 48 kcal) costs, for example, about 3 € (62.50 € for 250 g; there are also sources that are cheaper by a factor of 2 to 3, but the problems mentioned above still remain) at the company Falcento (other suppl.[30] The annual dose recommended in the article would therefore cost just over € 1000. However, the same caloric amount of keto-genic medium-chain fatty acids from coconut oil (5.5 g medium-chain fatty acids with about 9.1 kcal/g in about 10 g coconut oil, i.e. also about 50 kcal) costs only about 10 cents, and you can even get them in organic quality. With an annual dose of only about €35, healthy coconut oil is much cheaper than unhealthy galactose. In case of hippocampal insulin resistance, however, I recommend up to 50 g of coconut oil per day, as a butter substitute for baking, frying or as a spread on bread (see my book “Kopfküche”), which with about 450 kcal already covers almost a quarter of the entire basic energy requirement for about 50 cents per day. Healthy eating can hardly be more cost-effective.
Summary
The idea of overcoming impaired glucose uptake due to hippocampal insulin resistance in Alz-heimer’s disease by administering galactose is not scientifically plausible. Galactose is not a suit-able substitute for glucose, on the contrary, as shown in animal experiments. Only ketone bod-ies, which are produced by the body from long-chain fatty acids during overnight fasting and from medium-chain fatty acids, such as those found in coconut oil, during non-fasting, are suita-ble as natural substitutes. Compared to galactose, which is pro-inflammatory and therefore det-rimental to health, ketone bodies have the additional property of stimulating the formation of new brain cells and rejuvenating neurons, in addition to providing a natural energy supply. The natural way to bypass hippocampal insulin resistance and energize the autobiographical memory center is to produce ketone bodies by fasting at night, avoiding blood sugar spiking foods, and using comparatively inexpensive coconut oil.
Sources:
[1] de la Monte SM: Type 3 diabetes is sporadic Alzheimer׳s disease: mini-review. Eur Neuropsychopharmacol 2014, 24:1954-1960, https://www.ncbi.nlm.nih.gov/pmc/articles/PMC4444430/
[2] Marcus C et al: Brain PET in the diagnosis of Alzheimer’s disease. Clin Nucl Med 2014, 39:413-426, https://www.ncbi.nlm.nih.gov/pmc/articles/PMC4332800/
[3] Michailidis M et al: Alzheimer’s Disease as Type 3 Diabetes: Common Pathophysiological Mechanisms between Alzheimer’s Disease and Type 2 Diabetes. Int J Mol Sci. 2022, https://www.ncbi.nlm.nih.gov/pmc/articles/pmid/35269827/
[4] Planchez B et al: Increasing Adult Hippocampal Neurogenesis Promotes Resilience in a Mouse Model of Depression. Cells 2021, https://pubmed.ncbi.nlm.nih.gov/33919292/; Hill AS et al: Increasing Adult Hippocampal Neurogenesis is Sufficient to Reduce Anxiety and Depression-Like Behaviors. Neuropsychopharmacology 2015, 40: 2368-2378, https://www.nature.com/articles/npp201585
[5] Nehls M: Unified theory of Alzheimer’s disease (UTAD): implications for prevention and curative therapy. J Mol Psychiatry 2016, https://www.ncbi.nlm.nih.gov/pmc/articles/pmid/27429752/
[6] Thomas R et al: Exercise Training Results in Lower Amyloid Plaque Load and Greater Cognitive Function in an Intensity Dependent Manner in the Tg2576 Mouse Model of Alzheimer’s Disease. Brain Sci 2020, https://pubmed.ncbi.nlm.nih.gov/32046299/; He XF et al: Voluntary Exercise Promotes Glymphatic Clearance of Amyloid Beta and Reduces the Activation of Astrocytes and Microglia in Aged Mice. Front Mol Neurosci 2017, https://pubmed.ncbi.nlm.nih.gov/28579942/; https://www.nih.gov/news-events/nih-research-matters/sleep-deprivation-increases-alzheimers-protein (04/24/2018); Reddy OC & van der Werf YD: The Sleeping Brain: Harnessing the Power of the Glymphatic System through Lifestyle Choices. Brain Sci 2020, https://www.ncbi.nlm.nih.gov/pmc/articles/PMC7698404/
[7] Storhaug CL et al: Country, regional, and global estimates for lactose malabsorption in adults: a systematic review and meta-analysis. Lancet Gastroenterol Hepatol 2017, 2:738-746, https://pubmed.ncbi.nlm.nih.gov/28690131/
[8] Bo-Htay C et al: Effects of d-galactose-induced aging on the heart and its potential interventions. J Cell Mol Med 2018, 22:1392-1410, https://www.ncbi.nlm.nih.gov/pmc/articles/PMC5824366/
[9] Kenawy S et al: Involvement of insulin resistance in D-galactose-induced age-related dementia in rats: protective role of metformin and saxagliptin. PLoS One 2017, https://www.ncbi.nlm.nih.gov/pmc/articles/PMC5568415/
[10] Zhang Q et al: D-galactose injured neurogenesis in the hippocampus of adult mice. Neurol Res 2005, 27:552-556, https://pubmed.ncbi.nlm.nih.gov/15978184/; Cui X et al: Chronic systemic D-galactose exposure induces memory loss, neurodegeneration, and oxidative damage in mice: protective effects of R-alpha-lipoic acid. J Neurosci Res 2006, 83:1584-1590, https://pubmed.ncbi.nlm.nih.gov/16555301/
[11] Gao J et al: Salidroside suppresses inflammation in a D-galactose-induced rat model of Alzheimer’s disease via SIRT1/NF-κB pathway. Metab Brain Dis 2016, 31:771-778, https://pubmed.ncbi.nlm.nih.gov/26909502/; Li L et al: Moderate exercise prevents neurodegeneration in D-galactose-induced aging mice. Neural Regen Res 2016, 11:807-815, https://pubmed.ncbi.nlm.nih.gov/27335566/
[12] Li H, Zheng L, Chen C, Liu X, Zhang W. Brain Senescence Caused by Elevated Levels of Reactive Metabolite Methylglyoxal on D-Galactose-Induced Aging Mice. Front Neurosci 2019, https://www.ncbi.nlm.nih.gov/pmc/articles/PMC6760031/
[13] Gu Y et al: Food combination and Alzheimer disease risk: a protective diet. Arch Neurol 2010, 67:699-706, https://www.ncbi.nlm.nih.gov/pmc/articles/PMC3029147/
[14] Cotter DG et al: Obligate role for ketone body oxidation in neonatal metabolic homeostasis. J Biol Chem 2011, 286:6902-6910, https://www.ncbi.nlm.nih.gov/pmc/articles/PMC3044945/
[15] Robinson AM & Williamson DH: Physiological roles of ketone bodies as substrates and signals in mammalian tissues. Physiol Rev 1980,60:143-87, https://journals.physiology.org/doi/abs/10.1152/physrev.1980.60.1.143
[16] https://www.tz.de/leben/gesundheit/medizin-nobelpreis-zellforscher-yoshinori-ohsumi-erklaert-gesunde-ernaehrung-6806197.html (10/3/2016)
[17] Mattson MP et al: Intermittent metabolic switching, neuroplasticity and brain health. Nat Rev Neurosci 2018, 19:63-80, https://www.ncbi.nlm.nih.gov/pmc/articles/pmid/29321682/
[18] Maffezzini C et al: Metabolic regulation of neurodifferentiation in the adult brain. Cell Mol Life Sci 2020, 77:2483-2496, https://www.ncbi.nlm.nih.gov/pmc/articles/pmid/31912194/
[19] Gano LB et al: Ketogenic diets, mitochondria, and neurological diseases. J Lipid Res 2014, 55:2211-2228, https://www.ncbi.nlm.nih.gov/pmc/articles/pmid/24847102/
[20] Newman JC & Verdin E: Ketone bodies as signaling metabolites. Trends Endocrinol Metab 2014, 25:42-52, https://www.ncbi.nlm.nih.gov/pmc/articles/PMC4176946/
[21] Robinson AM & Williamson DH: Physiological roles of ketone bodies as substrates and signals in mammalian tissues. Physiol Rev 1980,60:143-87, https://journals.physiology.org/doi/abs/10.1152/physrev.1980.60.1.143
[22] Owen OE et al: Brain metabolism during fasting. J Clin Invest 1967, 46:1589-1595, https://www.ncbi.nlm.nih.gov/pmc/articles/PMC292907/
[23] Hertz L et al: Effects of ketone bodies in Alzheimer’s disease in relation to neural hypometabolism, β-amyloid toxicity, and astrocyte function. J Neurochem 2015, 134:7-20, https://pubmed.ncbi.nlm.nih.gov/25832906/
[24] Hilditch TP & Meara ML: Human-milk fat: 1. component fatty acids. Biochem J 1944, 38:29-34; Mazzocchi A et al: The Role of Lipids in Human Milk and Infant Formulae. Nutrients 2018, www.ncbi.nlm.nih.gov/pmc/articles/PMC5986447
[25] Hachey, DL et al: “Human lactation. II: Endogenous fatty acid synthesis by the mammary gland”, Pediatr Res 1989, 25:63-68.
[26] Cunnane, SC & Crawford, MA: ‘Energetic and nutritional constraints on infant brain development: implications for brain expansion during human evolution’, J Hum Evol 2014, 77:88-98.
[27] Taylor, MK et al: “Dietary Neuroketotherapeutics for Alzheimer’s Disease: An Evidence Update and the Potential Role for Diet Quality,” Nutrients 2019, www.ncbi.nlm.nih.gov/pmc/articles/PMC6722814
[28] Jung WK et al: Advanced Glycation End Products Increase Salivary Gland Hypofunction in d-Galactose-Induced Aging Rats and Its Prevention by Physical Exercise. Curr Issues Mol Biol. 2021, 43:2059-2067, https://www.ncbi.nlm.nih.gov/pmc/articles/PMC8928989/
[29] Westphal-Lanzenberger L: Galactose: Intelligent sugar against Alzheimer’s disease and insulin resistance. 2023. 4:23-29
[30] https://produkte.falcento-shop.com/epages/es10636382.sf/de_DE/?ObjectPath=/Shops/es10636382/Categories/Galactose (last accessed 7/07/2023)
Here you can find out which nutritional concept is more promising:
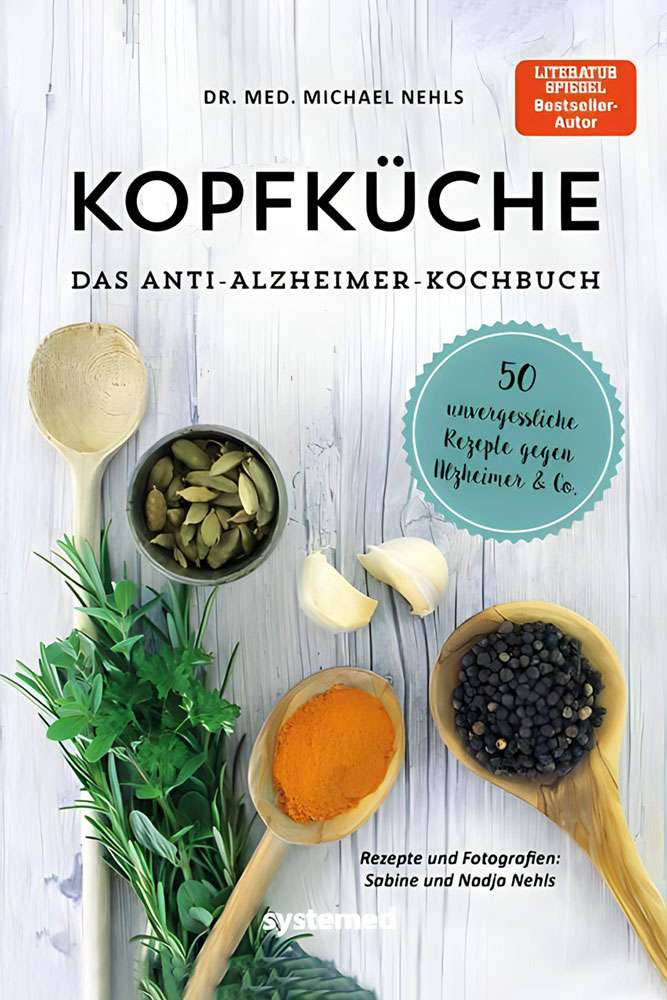